Study of the behavior and determination of phenol Based on modified carbon pa s te electrode with nickel oxide-nitrogen carbon quantum dots using cyclic voltammetry
Volume 6, Issue 04, Pages 58-68, March 2023 *** Field: Analytical Environmetal Chemistry
Abstract
The behavior of phenol was studied and determined using the modified carbon paste electrode (MCPE) with nickel oxide nanoparticles doped by nitrogen carbon quantum dots as nanoadsorbent (NiO - NCQD) and cyclic voltammetry (CV). The MCP electrode was manufactured in a laboratory. The modified carbon paste consisted of 12% (NiO-NCQD), 44% of graphite powder and 44% of paraffin oil to get a modified carbonate paste. Cyclic voltammetry can provide behavior information; as such: diffusion coefficient (D), charge transfer coefficient (α.nα), the mass transport (mtrans) found that diffusion coefficient, the reduction of mass transport (mtrans) by increasing the phenol concentration in the solution, and increasing of constant K0 when the concentration of phenol increased in the solution. Also, the highest occupied molecular orbital (HOMO), lowest unoccupied molecular orbital (LUMO), and Gibbs free energy (ΔG) are studied and calculated. In this study, EHOMO=4.92eV, ELUMO=0.32eV, and ΔG=-4.17 were considered. The drinking water samples from Latakia city were analyzed based on NiO-NCQD adsorbent using the MCPE method (NiO-NCQD/MCPE). The phenol concentration in the drinking water sample in Latakia was achieved less than the quantitative detection limit (LOQ), and the proposed procedure was validated by spiking samples.
References
M. Alizadeh, P. Nasehi, M.S. Moghaddam, S. Agarwal, V.K. Gupta, Electrochemical sensing of phenol in different water sources by a titanium oxide nanotubes/single-wall carbon nanotubes nanocomposite-ionic liquid amplified sensor, Int. J. Electrochem. Sci., 16 (2021) 210774. https://doi.org/10.20964/2021.06.6
A. Abd Gami, M.Y. Shukor, K.A. Khalil, F.A. Dahalan, A. Khalid, S.A. Ahmad, (2014). Phenol and its toxicity, J. Environ. Microbiol. Toxicol., 2 (2014) 11-23. https://doi.org/10.54987/jemat.v2i1.89
M. Chand Meena, R. Band, G. Sharma, Phenol and its toxicity: A case report, Iran. J. Toxicol., 8 (27) (2015) 1222-1224. http://ijt.arakmu.ac.ir/article-1-383-en.html
A. Tyagi, S. Tyagi, N. Malik, H. Chawla, Suicidal phenol ingestion: A case report, Int. J. Forensic Med. Toxicol. Sci., 2021, 2, 1: 22-23. https://www.academia.edu/download/54620082/IJFMTS_21_22-23.pdf
W.W. Anku, M.A. Mamo, P.P. Govender, Phenolic compounds in water: sources, reactivity, toxicity and treatment methods, Chapter 17, (2017) 419-443. http://dx.doi.org/10.5772/166927
A.O. Adeola, Fate and toxicity of chlorinated phenols of environmental implications: a review, Med. Anal. Chem. Int. j., 2 (4) (2018) 000126. http://doi.org/10.23880/macij-16000126
R.M. Bruce, J. Santodonato, M.W. Neal, Summary review of the health effects associated with phenol, Toxicol. Ind. Health, 3 (4) (1987) 535-568. https://doi.org/10.1177/074823378700300407
J. Michałowicz, W. Duda, (2007). Phenols-sources and toxicity, Polish J. Environ. Stud., 16 (3) (2007) 347–362. http://www.pjoes.com/Phenols-Sources-and-Toxicity,87995,0,2.html
D. Ferraz, D.V. Thomaz, R.S. Antunes, F.M. Lopes, (2021). Development of a low-cost colorimetric paper-based spot test for the environmental monitoring of phenolic pollutants, Environ. Challenges, 4 (2021) 100128. https://doi.org/10.1016/j.envc.2021.100128
A. Mulyasuryani, A.M. Mustaghfiroh, Development of potentiometric phenol sensors by Nata de coco membrane on screen-printed carbon electrode, J. Anal. Methods Chem., 2019 (2019) 4608135. https://doi.org/10.1155/2019/4608135
N.G. Simões, V.V. Cardoso, E. Ferreira, M. J. Benoliel, C.M. Almeida, Experimental and statistical validation of SPME-GC–MS analysis of phenol and chlorophenols in raw and treated water. Chemosphere, 68 (2007) 501-510. https://doi.org/10.1016/j.chemosphere.2006.12.057
O. Folin, V. Ciocalteu, On tyrosine and tryptophane determinations in proteins. J. Biol. Chem, 73 (1927) 627-650. https://doi.org/10.1016/S0021-9258(18)84277-6
A. Blainski, G.C. Lopes, J.C.P. De Mello, Application and analysis of the folin ciocalteu method for the determination of the total phenolic content from Limonium brasiliense L, Molecules, 18 (2013) 6852-6865. https://doi.org/10.3390/molecules18066852
C. Priyanthi, R. Sivakanesan, The total antioxidant capacity and the total phenolic content of rice using water as a solvent, Int. J. Food Sci., 2021 (2021) 1-6. https://doi.org/10.1155/2021/5268584
D. Bajčan, L. Harangozo, D. Hrabovská, D. Bončíková, Optimizing conditions for spectrophotometric determination of total polyphenols in wines using Folin-Ciocalteu reagent, J. Microbiol. Biotechnol. Food Sci., (2021) 1271-1280. https://www.jmbfs.org/
N. Siddiqui, A. Rauf, A. Latif, Z. Mahmood, Spectrophotometric determination of the total phenolic content, spectral and fluorescence study of the herbal Unani drug Gul-e-Zoofa (Nepeta bracteata Benth), J. Taibah Uni. Med. Sci., 12 (2017) 360-363. https://doi.org/10.1016/j.jtumed.2016.11.006
M. González, B. Guzman, R. Rudyk, E. Romano, M.A. Molina, Spectrophotometric determination of phenolic compounds in propolis, Acta Farmaceutica Bonaerense, 22 (2003) 243-248. http://portalrev.enfermagem.bvs.br/index.php?issn=0326-2383〈=en
V.L. Singleton, R. Orthofer, R.M. Lamuela-Raventós, Analysis of total phenols and other oxidation substrates and antioxidants by means of folin-ciocalteu reagent, Meth. Enzymol., 299 (1999) 152-178. https://doi.org/10.1016/S0076-6879(99)99017-1
K.C. Guimarães, D.L. Salgado, E.E.N. Carvalho, Evaluation of different methodologies for the determination of phenolic compounds in tropical fruits, Brazil. J. Food Technol., 23 (2020) 01519. https://doi.org/10.1590/1981-6723.01519
X. Chen, Y. Liang, X. Zhou, Y. Zhang, Phenol removal by a novel non-photo-dependent semiconductor catalyst in a pilot-scaled study: effects of initial phenol concentration, light, and catalyst loading, J. Nanomaterials., 2014 (2014) 1-8. https://doi.org/10.1155/2014/457485
K. Nakashima, S. Kinoshita, M. Wada, N. Kuroda, W.R. Baeyens, HPLC with fluorescence detection of urinary phenol, cresols and xylenols using 4-(4, 5-diphenyl-1 H-imidazol-2-yl) benzoyl chloride as a fluorescence labeling reagent, Analyst, 123 (1998) 2281-2284. https://doi.org/10.1039/A804582H
M.D.P. Fernández-Poyatos, A. Ruiz-Medina, C. Salazar-Mendías, E.J. Llorent-Martínez, Spectrophotometric determination of the antixidant properties and characterization of the phenolic content by high-performance liquid chromatography–diode array detection–tandem mass spectrometry (HPLC–DAD–MS/MS) of Berberis hispanica Boiss. & Reut. Leaves, Anal. Lett., 54(4) (2021) 646-657. https://doi.org/10.1080/00032719.2020.1775628
A.S. Adekunle, O.A. Arotiba, B.O. Agboola, N.W. Maxakato, B.B. Mamba, (2012). Voltammetric and impedance studies of phenols and its derivatives at carbon nanotubes/prussian blue films platinum modified electrode, Int. J. Electrochem. Sci., 7 (2012) 8035-8051. https://core.ac.uk/download/pdf/54194818
T.A. Enache, A.M. Oliveira-Brett, (2011). Phenol and para-substituted phenols electrochemical oxidation pathways, J. Electroanal. Chem., 655 (2011) 9-16. https://doi.org/10.1016/j.jelechem.2011.02.022
K.J. Lee, B.D. McCarthy, J.L. Dempsey, On decomposition, degradation, and voltammetric deviation: the electrochemist's field guide to identifying precatalyst transformation, Chem. Soc. Rev., 48 (11) (2019) 2927-2945. https://doi.org/10.1039/C8CS00851E
[ A. Habekost, Simulation and fitting of cyclic voltammetry and chronoamperometry curves of electrochemical reactions with different mechanisms—A didactic perspective, World J. Chem. Educ., 7 (2019) 53-64. https://doi.org/10.12691/wjce-7-2-4
M.C. Henstridge, E. Laborda, E.J. Dickinson, R.G. Compton, Redox systems obeying Marcus–Hush–Chidsey electrode kinetics do not obey the Randles–Ševčík equation for linear sweep voltammetry, J. Electroanal. Chem., 664 (2012) 73-79. https://doi.org/10.1016/j.jelechem.2011.10.015
J.E. Randles, A cathode ray polarograph. Part II, the current-voltage curves, Trans. Faraday Soc., 44 (1948) 327-338. https://doi.org/10.1039/TF9484400327
A. García-Miranda Ferrari, C.W. Foster, P.J. Kelly, D.A. Brownson, C.E. Banks, Determination of the electrochemical area of screen-printed electrochemical sensing platforms, Biosens., 8 (2018) 53. https://doi.org/10.3390/bios8020053
Y. Uchida, E. Kätelhön, R.G. Compton, (2018). Cyclic voltammetry with non-triangular waveforms: Electrochemically irreversible and quasi-reversible systems, J. Electroanal. Chem., 810 (2018) 135-144. https://doi.org/10.1016/j.jelechem.2017.12.053
N. Aristov, A. Habekost, Cyclic voltammetry-A versatile electrochemical method investigating electron transfer processes, World J. Chem. Edu., 3 (2015) 115-119. https://doi.org/10.12691/wjce-3-5-2
A. Oleinick, I. Svir, C. Amatore, Transient cyclic voltammetry: new theoretical challenges to bring up to date a famous electrochemical lady, J. Solid State Electrochem., 24 (2020) 2023-2025. https://link.springer.com/article/10.1007/s10008-020-04553-x
M. Favaro, Stochastic analysis of electron transfer and mass transport in confined solid/liquid interfaces, Surf., 3 (2020) 392-407. https://doi.org/10.3390/surfaces3030029
S.M. Sayyah, M. Shaban, A.B. Khaliel, M. Rabia, Electropolymerization of m-cresol on platinum electrode from aqueous acidic solution, Int. J. Chem., 35 (2014) 2051-2732. https://www.ccsenet.org/journal/index.php/ijc
J. J.Y. Wong, Electrochemical focal hypoxia for cell models, Ph.D. thesis, Department of Chemical Engineering and Biotechnology, University of Cambridge, 2021. https://doi.org/10.17863/CAM.75017
R. Pakkath, E.K. Reddy, S. Kuriakose, C. Saritha, A.M. Sajith, R.P. Karuvalam, K.R. Haridas, K. R. Synthesis, characterization and determination of HOMO-LUMO of the substituted 1, 3, 5-triazine molecule for the applications of organic electronics, J. Korean Chem. Soc., 63 (2019) 352-359. https://doi.org/10.5012/jkcs.2019.63.5.352
J. Ebrahimian, M. Khayatkashani, N. Soltani, Q.A. Yousif, M. Salavati-Niasari, Catechin mediated green synthesis of Au nanoparticles: Experimental and theoretical approaches to the determination HOMO-LUMO energy gap and reactivity indexes for the (+)-epicatechin (2S, 3S), Arab. J. Chem., 15 (2022) 103758. https://doi.org/10.1016/j.arabjc.2022.103758
Y.C. Chen, Y.T. Kuo, T.H. Ho, Photo-polymerization properties of type-II photoinitiator systems based on 2-chlorohexaaryl biimidazole (o-Cl-HABI) and various N-phenylglycine (NPG) derivatives, Photochem. Photobiol. Sci., 18 (2019) 190-197. https://link.springer.com/article/10.1039/c8pp00300a.
H. Zhang, L. Yuan, X. Tang, J. Hu, J. Sun, Y. Zhang, R. Jia, Influence of metal gate electrodes on electrical properties of atomic-layer-deposited Al-rich HfAlO/Ga2O3 MOSCAPs. IEEE Trans. Electron Devices, 67 (2020) 1730-1736. https://doi.org/ 10.1109/TED.2020.2974794
G.K. Jayaprakash, B.K. Swamy, S. Rajendrachari, S.C. Sharma, R. Flores-Moreno, Dual descriptor analysis of cetylpyridinium modified carbon paste electrodes for ascorbic acid sensing applications. J. Mol. Liq., 334 (2021) 116348. https://doi.org/10.1016/j.molliq.2021.116348
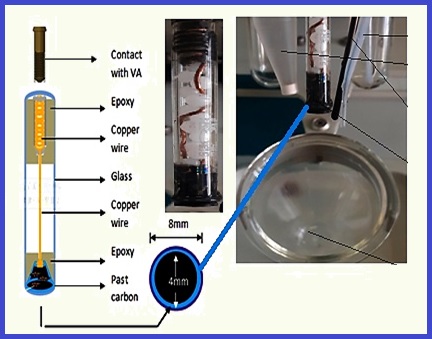
Copyright (c) 2023 Analytical Methods in Environmental Chemistry Journal

This work is licensed under a Creative Commons Attribution 4.0 International License.
JOURNAL PUBLISHING AGREEMENT
PLEASE PROVIDE US THE FOLLOWING INFORMATION,
Article entitled:
Corresponding author:
To be published in the journal:
Your Status
I am the sole author of the manuscript
- I am an Iranian government employee.
- I am a European government employee
- I am a Asian government
- None of the above
I am one author signing on behalf of all co-authors of the manuscript
- I am an Iranian government employee.
- I am a European government employee
- I am a Asian government
- None of the above
Please tick the above blanks (as appropriate), review the Journal Publishing Agreement, and then sign and date the document in black ink.
Published Journal Article: the author may share a link to the formal publication through the relevant DOI. Additionally theses and dissertations which contain embedded Published Journal Articles as part of the formal submission may be hosted publicly by the awarding institution with a link to the formal publication through the relevant DOI. Any other sharing of Published Journal Articles is by agreement with the publisher only.
Signed: ______________________________________ Name printed: ___________________________________________
Title and Company (if employer representative): _______________________Date: __________________________________